How Light Tells Us the Story of the Universe
Length: • 7 mins
Annotated by howie.serious
6 min read
Almost everything we know about the cosmos is conveyed by photons traveling across vast distances
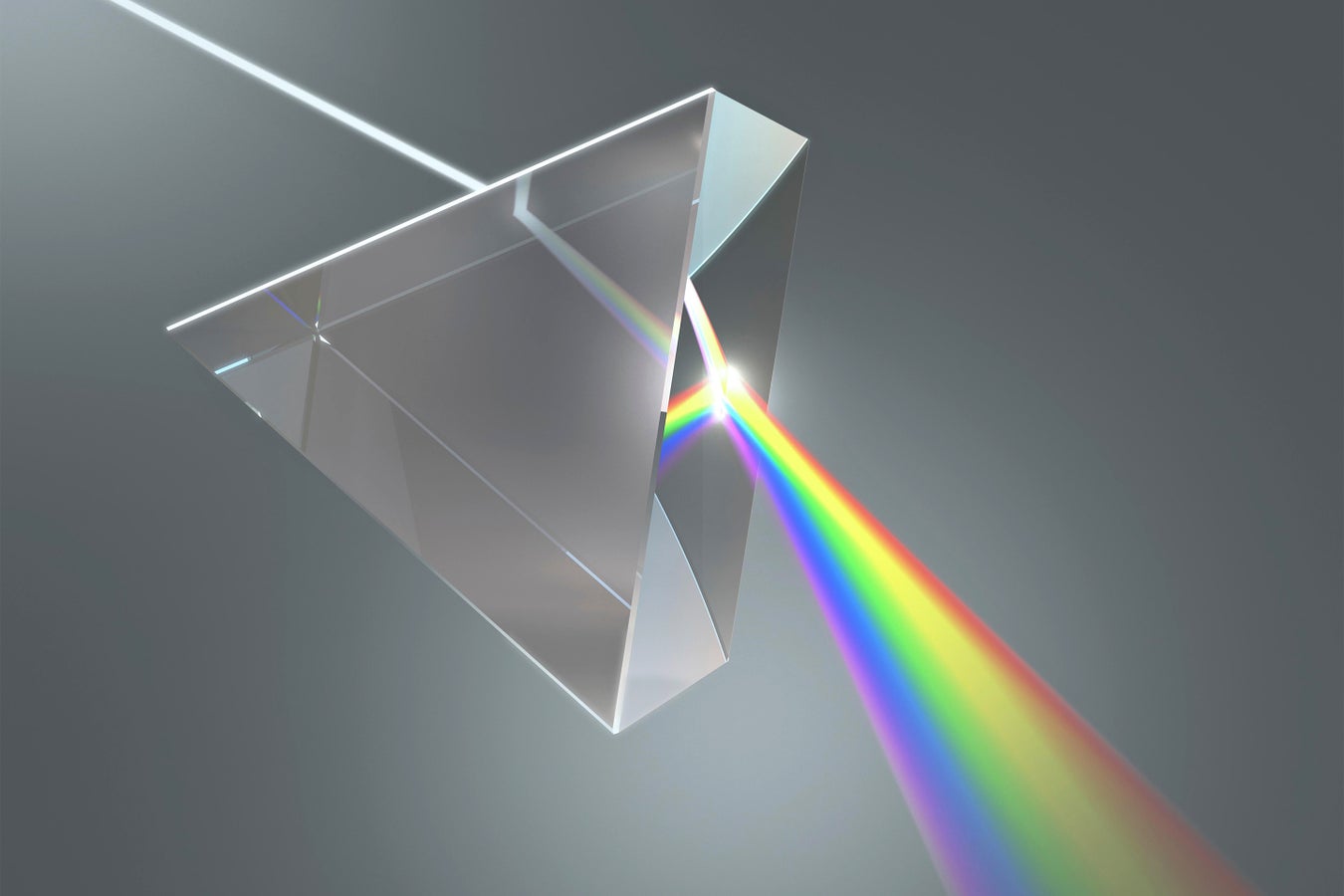
Photons are odd little beasts.
They can act like waves. They can act like particles. They are teeny tiny messengers of force. They are carriers of energy.
But most of all, they’re light. When you think of light, you’re thinking of photons. So literally, when you look around you and see, well, anything, your eyes are detecting photons that are emitted by objects like your computer screen or a lamp, photons emitted by those sources that are reflected off other objects or an absence of photons—a result of something absorbing or blocking them as they travel through space. Because of this, nearly everything we know about objects in deep space is because of light.
Another thing photons are is weird—very, very weird.
In many ways they behave like waves, similar to those on the beach or in your bathtub when you splash around, with crests and troughs. The distance between crests is called the wavelength, and the amplitude of a wave—its strength, effectively—is the difference in height between the trough and a crest. In a sound wave, that’s related to the volume of the sound. And in light, it’s related to the light’s intensity.
In other ways, photons act like subatomic particles, which can have momentum, spin, and more. It’s very difficult to wrap your head around the idea that light can be a wave and a particle, even at the same time, but quantum mechanics is exceptionally bizarre that way (which is a big reason it took so long to be accepted by scientists as a good model of reality). These properties, however, define light, and they in turn tell us a lot about the objects that emit or reflect it.
For light, the most fundamental property is the wavelength. That describes how much energy the light has, with shorter wavelength waves having more energy than ones with longer wavelengths. More colloquially, we see this difference in wavelength (or energy) as color. When you see something as violet, for example, you’re seeing light coming from that object with a shorter wavelength. Blue has a slightly longer wavelength, green longer again, then yellow, orange and finally red, with the longest we see. We can measure the wavelengths of these colors of visible light to determine the range our eyes can detect, and it goes very roughly from 380 nanometers (nm) for violet to about 750 nm for red. (One nanometer is a billionth of a meter.)
An aside: the frequency of light is another fundamental property and is a measure of how frequently the crests pass an observer. It’s equal to the inverse of the wavelength; in other words, the longer the wavelength, the lower the frequency, and vice versa. Scientists use both, usually picking one or the other in their calculations depending on what makes the math easier or more intuitive.
Our eyes have evolved cells called cones in our retinas that are sensitive to different wavelengths of light. There are three kinds: one detects a small range of wavelengths centered on red, and the others detect ranges centered on green and blue. As light hits those cells, they send signals to the brain, which combines them to create the colors we see.
Light can have wavelengths well outside what our eyes can see, too. Scientist William Herschel figured this out in the 19th century, when he found he could still feel heat from sunlight even when it was passed through dark filters. He had discovered infrared light, with wavelengths greater than 750 nm. Beyond that, at progressively longer wavelengths, are microwaves, millimeter waves and radio waves. On the other end of the spectrum there’s ultraviolet, with wavelengths shorter than what we see as violet. Progressively shorter still are x-rays and then gamma rays, the latter with wavelengths shorter than about 10 picometers (or one one-hundredth of a nanometer).
Because energy scales inversely with wavelength (or directly with frequency, if you prefer), gamma rays have a lot of energy, while radio waves have very little. As a category, gamma rays include any light with wavelengths less than those of x-rays (or an energy higher than x-rays). Radio waves have an open-ended range as well but at the long wavelength end of the spectrum; a wavelength measured in light-years would still be considered a radio wave.
And this brings us to the universe. Excluding meteorites, which fall to the ground and can be examined, until recently all the information we got from the cosmos outside our atmosphere was from light. Stars, galaxies, dust clouds, exoplanets: all of these emit or reflect or absorb light, creating signals that travel across space for incredible distances to be detected here on Earth.
And those signals—that light—tell us the story of the universe.
For example, hot objects glow with their own light. They emit a wide range of colors, but at a given temperature there’s a peak wavelength where it emits the most light. That peak wavelength gets shorter as temperatures get hotter. Stars like the sun emit light most strongly in the visible spectrum. That’s no coincidence; there’s so much sunlight around that it’s a very handy tool to get information about your environment, which is why so many creatures on Earth evolved eyes to detect it. Cooler stars peak more in the red, hotter ones in the blue, and that’s why stars have colors.
Just by measuring a star’s color you can tell its temperature. That’s an amazing thing—taking the temperature of an object that can be quintillions of kilometers away!
That temperature also tells us about the physics behind the light. It takes a lot of energy to make x-rays, and even more to make gamma rays, so if we see them coming from an object, we know it packs a punch (and usually has extremely strong magnetic fields, which can supercharge subatomic particles and give them the energy needed to emit these kinds of light). Neutron stars, black holes, intergalactic gas clouds colliding, even tangled magnetic fields on the sun, can all blast out x- and gamma rays, indicating these objects’ true power.
For visible light (as well as ultraviolet and infrared), astronomers use filters to determine colors to measure stellar temperatures, among other things. These filters can be, for example, treated glass plates that allow a narrow range of wavelengths of light to pass through them so they can isolate colors like blue, green or red.
But we can do much better. Passing light through a prism or over a grating—a piece of glass or metal that has a series of very fine parallel grooves in it—breaks up light into many individual colors. The resulting spectrum, as it’s called, can have thousands of individual wavelengths detectable in it. And this ability is key to understanding the universe. And for once, I’m not exaggerating even a little bit.
Different atomic elements absorb and emit light at very specific colors (the electrons zipping around the atom’s nucleus can only absorb discrete amounts of energy, a fact that gave rise to the development of quantum mechanics in the first place). Hydrogen, for example, strongly absorbs light at 656 nm (in the red). Some stellar spectra show that as a deep dark band or line at that wavelength in the light they emit. This is how astronomers first determined stars are mostly made of hydrogen. But often we see absorption lines for many different elements at different wavelengths, allowing us to determine more thoroughly what stars are made of; this technique can also tell us what elements are in gas clouds and even the atmospheres of exoplanets orbiting other stars.
And there’s so much more. If an object is moving toward you, the wavelengths of the light it emits get compressed, similar to how sound waves from an approaching ambulance’s siren will be compressed and rise in pitch. For light, we call this a blueshift. If an object is moving away, the wavelengths of its light instead get stretched, called redshifting. Stars and galaxies rotate, shifting their light in a way that allows astronomers to determine how rapidly they’re spinning. Moreover, in the early 20th century astronomers figured out that more distant galaxies have larger redshifts, meaning the universe is expanding. By precisely measuring these redshifts they could also figure out how rapidly the cosmos is expanding as well—and how long it’s been doing so, giving us its age of 13.8 billion years.
I mentioned light was the only way we would get information from space until recently. Now we can also detect other sorts of cosmic messengers: subatomic particles such as neutrinos and cosmic rays and other phenomena such as gravitational waves, literally ripples in the fabric of spacetime. We can combine that information with the light we detect, meaning we’re now in the era of “multimessenger” astronomy, a powerful tool to help us understand what’s going on over our head.
So remember all this when you go outside on a clear night. The light you see from those stars twinkling above you traveled hundreds of trillions of kilometers at a ridiculous speed for years or decades, passing through space and Earth’s atmosphere and then finally being stopped by and absorbed by the cells in your eye. Light is a way for the universe to literally touch you. The least we can do is use that light to learn as much as we can about it.
Phil Plait is a professional astronomer and science communicator in Virginia. He writes the Bad Astronomy Newsletter. Follow him online.