A Manhattan Project for the climate
Length: • 15 mins
Annotated by Marc Chasserot
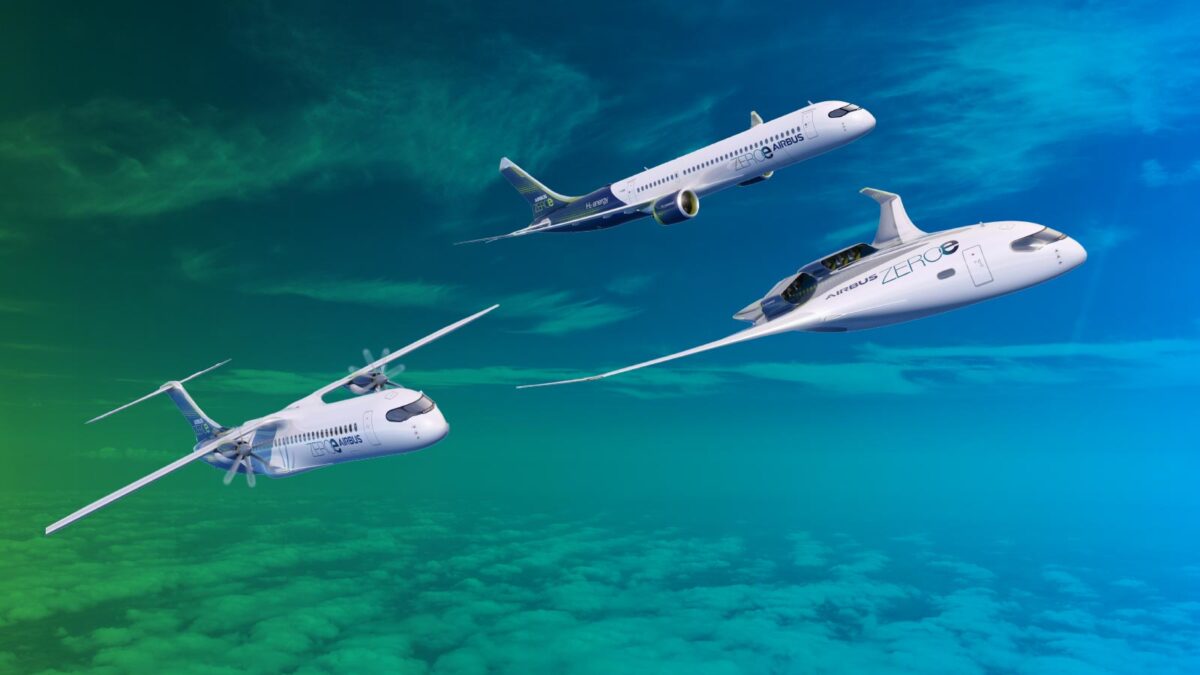
Even if a new breed of aircraft powered by hydrogen were available by 2035, that might not be fast enough to help much with the industry’s goal of reaching net-zero carbon emissions by 2050. Paul Marks takes us inside the technical challenges facing hydrogen aircraft — and the case for vastly increasing the resources currently applied to the necessary technologies and infrastructure.
★ Click at right to open this story’s Knowledge Guide entries.
Earlier this year, two budget airlines in India and one in Ireland placed a series of colossal orders for kerosene-fueled jetliners. These 1,270 aircraft contributed to the aviation industry’s best sales in the first half of any year since 2010, but the early 2030s delivery dates for many of the aircraft means they will be flying, and emitting climate-warming carbon dioxide, well past the middle of the century. That’s the very time period when the Montreal-based International Air Transport Association, whose member airlines carry 83% of the world’s passengers and cargo, wants to achieve net-zero carbon dioxide emissions for air travel, specifically by 2050.
How can net-zero be possible with so many kerosene-burning jets on order now? IATA has an answer, but it’s one that hydrogen fans aren’t convinced is feasible: IATA is encouraging the introduction of sustainable aviation fuels, SAF, made from renewable feedstocks like recycled cooking oils, agricultural waste and forestry debris. These fuels, which are just starting to be used, can be “dropped in” existing aircraft and burned just like fossil fuels. Today, they are blended with kerosene, but the aim is to fly aircraft on 100% SAF. No additional CO2 would be added to the atmosphere, because as the crops grew, they extracted CO2 to make their biomass.
In a rival approach, Airbus has teamed with CFM International, while Rolls-Royce has teamed with easyJet, a budget airline in the United Kingdom, to get hydrogen-powered aircraft onto the market by 2035. Even so, IATA doesn’t expect enough of those to be flying in time to make a major contribution to the net-zero goal. In fact, it places hydrogen combustion and hydrogen fuel cell options as only part of the 13% contribution it anticipates from “new technologies.” IATA expects the lion’s share of the contribution (65%) to be made by SAF. The balance of the way to net-zero would be achieved by capturing carbon from the air, planting additional trees to “offset” CO2 emissions and tightening flight routes, among other operational efficiencies.
If the aviation industry is to get hydrogen airliners flying sooner than 2035, what’s needed is a focused research and development effort akin to J. Robert Oppenheimer’s Manhattan Project, says Rob Miller, a professor of aerothermal technology and director of the Whittle Laboratory at the University of Cambridge in the U.K. Why might a project on such a scale be needed? The tempest of wildfires, heatwaves and other severe weather this year suggests we’re rapidly approaching the 1.5 degree Celsius threshold that could have catastrophic consequences, including severe flooding prompted by meters of sea level rise.
Founded by turbojet engine inventor Sir Frank Whittle, the Whittle Lab is charged with leading the U.K.’s research on zero-carbon flight, which it accomplishes in part by “silo busting” — encouraging disparate research organizations to work together to get results all the faster. To do this, Miller leads what he calls an “agile, multidisciplinary Skunk Works” at the lab, working to develop advanced hydrogen-fueled jet engines alongside engineers from Rolls-Royce, the University of Oxford and engineers from a host of other companies with relevant specialties.
It’s an approach he’d like to see global hydrogen researchers adopt. “If we had a more warlike spirit and we really focused and said, ‘We’re going to do this,’ I think we could really tighten up the timescales to hydrogen jet aviation,” Miller says.
As for the goal of flying hydrogen aircraft in 2035, “I think that’s doable, but I don’t think we’re going to get much earlier than that,” he adds, unless we have a Manhattan-scale project. Currently, hydrogen aircraft research is spread across multiple distinct domestic and pan-regional efforts. Among them is the European Union’s 27-nation, 654 million-euro Clean Aviation program, which includes the German Aerospace Center’s Ultra Performance Wing project, in which researchers are developing an efficient airliner wing for hydrogen aircraft and another design for SAF aircraft. Other projects include the Airbus/CFM and Rolls-Royce/easyJet initiatives.
Credit: Graphic by Thor Design
One organization that could focus minds on hydrogen aviation to a Manhattan Project scale, Miller suggests, would be the U.K.’s Advanced Research and Invention Agency. ARIA was founded in January 2022 to pursue development of as-yet unspecified “high-risk, high-reward” ideas, much as DARPA does for the U.S. Defense Department.
And it’s not just the engines: He stresses that research must be expanded to include light, durable cryogenic fuel tanks, the valving systems for the hydrogen and the control systems.
For its part, the nascent ARIA says it’s too early to say what the organization will be focusing on technologically, as it is still in the process of hiring its “founding cohort of program directors,” says a spokesperson.
The climate challenge
Atmospheric scientists calculate that commercial aviation today is responsible for 5% of global radiative forcing, the trapping of heat in the atmosphere. CO2 emissions are responsible for about 2.5% of the forcing, and the balance comes from emissions of other greenhouse gases, nitrogen oxides, and also particulates and water vapor that turn into contrails and clouds. That 5% isn’t a lot right now, but the share could grow without advances in clean technologies. Other sectors are working to cut their emissions. Meanwhile, passenger numbers are projected to double or even triple by 2050, sending aviation’s share of emissions to anywhere from 10% to 37% of the global total, according to calculations by the Aviation Impact Accelerator, an offshoot of the Whittle Lab that develops computer models for sustainable flight researchers.
While Boeing is focused on SAF, and IATA is largely counting on it, no one can say for certain that enough SAF can be produced to meet the envisioned 65% contribution to net-zero. IATA estimates that about 449 billion liters of SAF will be needed annually by 2050 to meet that goal, but in 2022, just 300 million liters were produced. Liquid hydrogen combusted directly in jet engines or converted into electricity via fuel cells to drive motors, however, would automatically rid flights of CO2 emissions, as there’s no carbon in their chemistry to emit. So why exactly is it taking so long to develop these new aircraft?
“There’s three embedded challenges,” says Alan Newby, director of aerospace technology and future programs at Rolls-Royce in Derby, U.K., where the company is developing hydrogen jet turbine technology with a number of industrial partners. “First, there are the technical challenges in developing the engine and the airplane.”
Rolls is focusing on hydrogen combustion “because for any kind of reasonable range and size of airplane, you’re going to need to fuel it with liquid hydrogen. So you have all the attendant challenges of having cryogenic tanks on an airplane, and then managing cryogenic fuel all the way till it gets to the engine, and then burning it.”
“The second challenge is a lack of green hydrogen infrastructure,” he says, referring to the production of hydrogen through the electrolysis of water using only renewably sourced electricity. “Over 95% of the hydrogen produced today comes from fossil fuels, and so industry in general has got a massive challenge on its hands to develop a green hydrogen production industry. Even if I had a viable aircraft today, I still wouldn’t have a viable aviation system.”
On that supply point in particular, Newby supports Miller’s notion of a hydrogen-specific Manhattan Project. “If we’re going to move quicker, the industry needs to receive more funding, not just on the technology but on the hydrogen infrastructure as well.”
And the third challenge? “There’s some uncertainty, though we think it’s all positive, about the non-CO2 pollutants of hydrogen combustion and the water vapor and contrails produced. But we think that’s soluble, quite frankly.” (As will be seen ahead, researchers at ARPA-E in the U.S. think it’s a solvable problem too.)
Newby and Rolls-Royce are not alone in recognizing these challenges and attacking those that they can. Rival CFM International, the joint venture of GE in the U.S. and Safran in France, is teamed with Airbus on its ZEROe initiative to develop two kinds of hydrogen engines and assess four candidate jetliners that would burn hydrogen and/or generate electricity with fuel cells. Other organizations are also conducting research on enabling technologies, including the Whittle Lab, the U.K.’s Aerospace Technology Institute, and Cranfield University — focusing on everything from test rigs to combustors, fuel injectors, cryogenic pumps, on-aircraft fuel transport issues and fuel storage.
On Newby’s first point (the technical challenges of the aircraft and engines), one has to take into account the curious energy density and volumetric properties of liquid hydrogen. “A kilogram of hydrogen produces three times the energy of a kilogram of kerosene, which is great because aircraft are always weight restricted,” he says. “The downside is that the kilogram of liquid hydrogen takes up four times the volume of a kilogram of kerosene.”
And that has major implications for two key features of the airframe layout: where you put the fuel tanks and the passengers. “With hydrogen aircraft, the combination of the fuel and tanks is lighter than with kerosene,” Miller says. But that additional volume requires extra large tanks that must be vacuum insulated to keep the liquid hydrogen at cryogenic temperatures of minus 235 C. So “you have to move those tanks from your wings into the fuselage,” he says, referring to the fact that today’s kerosene-fueled jets store most of their fuel in wing tanks.
And that change requires a complete redesign. “You can’t just retrofit an existing aircraft with hydrogen jet engines since, if you did that, moving the tanks from the wings into the fuselage pushes loads of passengers out,” Miller says. “And so, the number of passengers you fly on your aircraft becomes really small.”
For which read: utterly uneconomic. This means novel fuselages must be designed to accommodate these vacuum-insulated cryogenic tanks, and that must be done without heavily impacting seat numbers.
That’s why, in its ZEROe project, Airbus is evaluating four concepts to determine which is feasible to bring to market by 2035. Two have pretty conventional-looking cylindrical fuselages — one for a twin hydrogen-combusting turbofan and one for a twin hydrogen-combusting turboprop. On both, the aircraft’s pressurized volume ends near the trailing edge of the wings, and the cryogenic liquid hydrogen tanks are placed in the unpressurized region behind the aft-pressure bulkhead.
If you’re wondering, the fact that jets cruise through frigid air at temperatures of minus 55 C is actually of little help.
“It will be cooler behind the rear-pressure bulkhead, but that won’t be significant in maintaining the hydrogen as a liquid,” explains Glenn Llewellyn, Airbus vice president for zero-emission aircraft, who has teams working on various aspects of hydrogen in France, Germany, Spain and the U.K. “We have to keep the hydrogen below minus 253 degrees Celsius across all flight altitudes and atmospheric temperatures. The insulation chosen will maintain the hydrogen as a liquid for a significant period of time on the ground, as well as during the flight, so airlines can operate the aircraft in a similar way to today.”
Airbus plans to assess liquid hydrogen and fuel cell propulsion by trying out the technologies on its ZEROe demonstrator, a modified A380. Credit: Airbus
In the third candidate, a blended wing body, Airbus places the turbofans in a shroud atop the wing, and the cryogenic fuel tanks sit beneath the wing, hidden inside the aerodynamic composite structure.
All three designs have a potential hybrid feature: By gasifying some of the liquid hydrogen, fuel cells might generate electricity to supplement the liquid hydrogen combustion, as well as provide each aircraft’s “nonpropulsive” electrical energy needs — powering undercarriage, control surfaces, cabin systems and avionics, for instance. But Llewellyn says it’s too early to say if that will happen.
“We are studying a number of architectures with separate and integrated electrical power systems. We will have to demonstrate the same level of redundancy and availability as on today’s aircraft, as a minimum. So it’s yet to be decided how we do that.”
With the fourth ZEROe candidate, Airbus is studying an even larger role for fuel cells: providing all of the power and propulsion. Multiple “pods” — each containing hydrogen fuel cells, a liquid hydrogen tank and an electric motor — would be arrayed along the aircraft’s wings, freeing up precious space in the fuselage. The total number of pods is yet to be determined, but four (two on each wing) and six (three on each wing) are among the configurations that Airbus is considering. The advantage of this design would be that pods could be swapped out as needed, making for easy refueling and maintenance, and that the design could easily be tailored for different flight ranges by changing the number of pods.
Start your engines
So much for the airframes — what about the engines? The Airbus fuel cell concept would be for medium-haul flights of up to 2,000 kilometers, but most of the other companies evaluating fuel cells energized by compressed hydrogen gas stored in noncryogenic tanks are targeting short-haul regional flights. And test flights are underway: ZeroAvia in the U.K. has flown a 19-seat Dornier-228 twin prop a number of times, as has California-based Universal Hydrogen with a 40-seat De Havilland Canada Dash 8-300. For safety of flight, both companies to date have replaced just one of their aircraft’s conventional turboprop drives with an experimental hydrogen drivetrain.
Newby, the Rolls executive, follows such flights with interest but maintains that “all the work we and others have done suggests the gas turbine is the ultimate power plant” for long-haul, large passenger airliners.
At Rolls-Royce, he says, engineers began their hydrogen jet quest with tests — fueled, they stress, with wave-power-derived “green” hydrogen — in which they converted a kerosene-burning turboprop gas turbine to hydrogen operation. To do this, they modified one of their AE 1200 jet engines from a Saab 2000 regional twin prop. “It’s very closely related to the engine on the C-130 Hercules,” Newby notes.
“We converted the fuel injectors and the fuel nozzles to run on gaseous hydrogen and basically tested whether we could start the engine, whether we could handle it and run it — and we did. It was a very, very successful test,” he says.
What Rolls found is that the turbomachinery in the engine does not have to change; it’s components like fuel injectors that do. “It’s not like you’ve got to rip the whole of the engine apart and change every component. Quite the opposite. The only bit that really had to change was the fuel injector. So that was a very interesting piece of learning,” says Newby.
Meanwhile, in the Airbus/CFM project, engineers are also finding that turbomachinery architecture can be similar, “requiring only combustion and fuel system adaptation for hydrogen,” says Llewellyn. “The igniter technology may not differ too greatly from a kerosene one, but injectors will have differences in design to cope with hydrogen’s fuel characteristics and to minimize non-CO2 emissions.”
Those non-CO2 emissions include oxides of nitrogen gases, or NOx, created when nitrogen and oxygen from the air react as the hydrogen burns.
Rolls’ next move will be to take one of its Pearl 15 jet turbines — which power the Mach-0.9-capable Bombardier Express 6500 business jet — and convert it to burn hydrogen. This will involve developing all the cryogenic fuel distribution systems that the engine will need around it. Plans call for conducting ground tests and later flying the modified engine in a demonstrator.
“We’ll be looking at the combustion of hydrogen: Can we burn it safely and successfully and manage the pollutants [like NOx] that come with it?” says Newby. “Second, can we move it around the engine, control something which is running on hydrogen, and pump liquid hydrogen at minus 253 degrees Celsius, for example. And third, can we package an engine with all the new heat exchangers that we will need, plus the new fuel system and pumps. There’s a whole engine packaging challenge to meet.”
Aiding this research is Rolls’ involvement in a number of collaborative research programs with its prime hydrogen jet engine project sponsor easyJet, plus partners Spirit AeroSystems of the U.S. and Reaction Engines of the U.K., the latter having a cryogenic heat-exchanger technology that was originally developed for Skylon, a putative single-stage-to-orbit, air-breathing spaceplane.
On the Airbus side, flight tests are also in the works. Plans call for installing a CFM-built hydrogen combustion engine onto a modified A380 test aircraft and flying it between 2026 and 2028, powered by four liquid hydrogen tanks in the fuselage. Comparable flights are also planned with an in-development fuel cell engine.
“Clean” flying?
It’s not just NOx from hydrogen jet engines that will need careful controlling. Like kerosene engines do today, hydrogen turbines would produce water vapor as a result of the chemistry of hydrogen combustion in air: two hydrogen molecules combining with one oxygen molecule to create a whole lot of heat, plus two water molecules. And when warm, moist air from the engine exhaust contacts the frigid air at cruise altitudes, ice crystals can form reflective condensation trails, or contrails. These in turn may form cirrus clouds that trap heat in the atmosphere through radiative forcing.
Atmospheric scientists have strong evidence that the climate impact of contrails from kerosene-fueled planes could be as much as that caused by the CO2 they emit — in other words, an additional 2.5% of global warming could be due to contrails. The finding first appeared in September 2020 in what has turned out to be a highly influential research paper written by a team of 21 scientists, led by David Lee of the U.K.’s Manchester Metropolitan University, in the journal Atmospheric Environment.
“Only about one in 20 [kerosene-fueled] flights produces a persistent contrail that forms into cirrus cloud,” says Miller of the Whittle Lab. “The CO2 that’s produced by the flight is in the atmosphere for hundreds of years, but the contrail is only there for a fraction of a day. But for those few hours, we think the climate impact of the contrail could be about the same as from the CO2 from the flight hanging around for 100 years.”
Rolls-Royce and British airline easyJet powered this modified Rolls-Royce AE 2100-A turboprop with liquid hydrogen fuel. The ground test, conducted late last year, was the first in a planned series toward the goal of flying a Rolls-Royce Pearl 15 turbofan modified to burn hydrogen. Credit: Rolls-Royce
If the brave, new world of hydrogen aviation were to produce a great deal of contrail-based climate warming — perhaps even more than kerosene jets produce — the point of switching to hydrogen would be lost, as Aerospace America reported earlier this year [“The dark side of green,” June 2023].
As a result, engineers want to measure just how the emissions from a hydrogen engine differ from those of a kerosene one. To find out, DLR, the German Aerospace Center, alongside Airbus, plans to undertake a series of live in-flight experiments in which two jet-powered sailplanes — one propelled by kerosene, the other by hydrogen — will be followed by a DLR plane brimming with atmospheric sensors. The data harvested should reveal the size, distribution and density of water vapor, contrail ice crystals, NOx levels and particulates, allowing scientists to make some actionable comparisons between kerosene and hydrogen jet engine contrail formation at different altitudes.
Help may be at hand, and from an unlikely source: artificial intelligence. The frequency and conditions surrounding contrail formation has given the Advanced Research Projects Agency-Energy in Washington, D.C., an idea: Could it be possible to recognize the combination of atmospheric and engine exhaust conditions necessary to produce contrails and cirrus clouds?
If the answer is yes, pilots could then be advised to change altitude or engine settings and effectively switch off the climate-harming contrail. Peter de Bock, formerly a principal scientist working on jet engines at GE Global Research in upstate New York, thinks so. In February, he established the $10 million, 18-month PRE-TRAILS research program to solicit proposals from academia and industry on ways aircraft — both kerosene and hydrogen fueled — can sense and avoid contrail generation.
Short for Predictive Real-time Emissions Technologies Reducing Aircraft Induced Lines in the Sky, PRE-TRAILs is “designed to set up a mechanism where we can, with 80% accuracy, predict whether an aircraft’s emissions at any point of flying will generate a cloud,” de Bock says. “But right now, according to the data, we are 90% wrong in predicting whether we’re going to make a cloud or not. So let’s fix that first. I want to get up to 80% right.”
ARPA-E is hoping that researchers come up with ways to harness multiple data sources to train a deep-learning artificial intelligence model to recognize the blizzard of conditions suspected of combining to forge a persistent contrail.
“This is a fuel-independent mechanism. Basically, you have to study the atmosphere and know what your engine is producing and then corroborate using observations and other mechanisms during [AI model] training time, whether it is generating cloud,” says de Bock. The deep-learning model, he says, could ingest data from satellites, weather stations, engine sensors, multispectral cameras and humidity sensors.
How does he envisage pilots being advised of a need to change altitude? “That’s up to the teams to decide. But currently, there is information on the turbulence radar that’s fed back to ground control — so there is actually a feedback loop there already. So they could start rerouting flights, perhaps. There’s many implementation options that can be explored.”
The PRE-TRAILS program’s aims were developed in association with Steven Barrett, an MIT aeronautics and astronautics professor, and his colleagues at the university’s Laboratory for Aviation and the Environment. They have been working with Delta Air Lines to begin identifying the airliner movements that can prevent contrail formation on live Delta flights.
Miller of the Whittle Lab is impressed with this line of research: “What’s important about this ARPA-E research, from the hydrogen point of view, is that it’s likely that by 2035 we will be able to switch off most contrails by clever flying,” says Miller.
If it works as expected, contrails from kerosene flights could be switched off, too, so even those airlines currently buying airliners in record numbers today will be able to instantly cut their climate impact. But whether it will take a latter-day Manhattan Project to get us there remains to be seen.
Related Topics
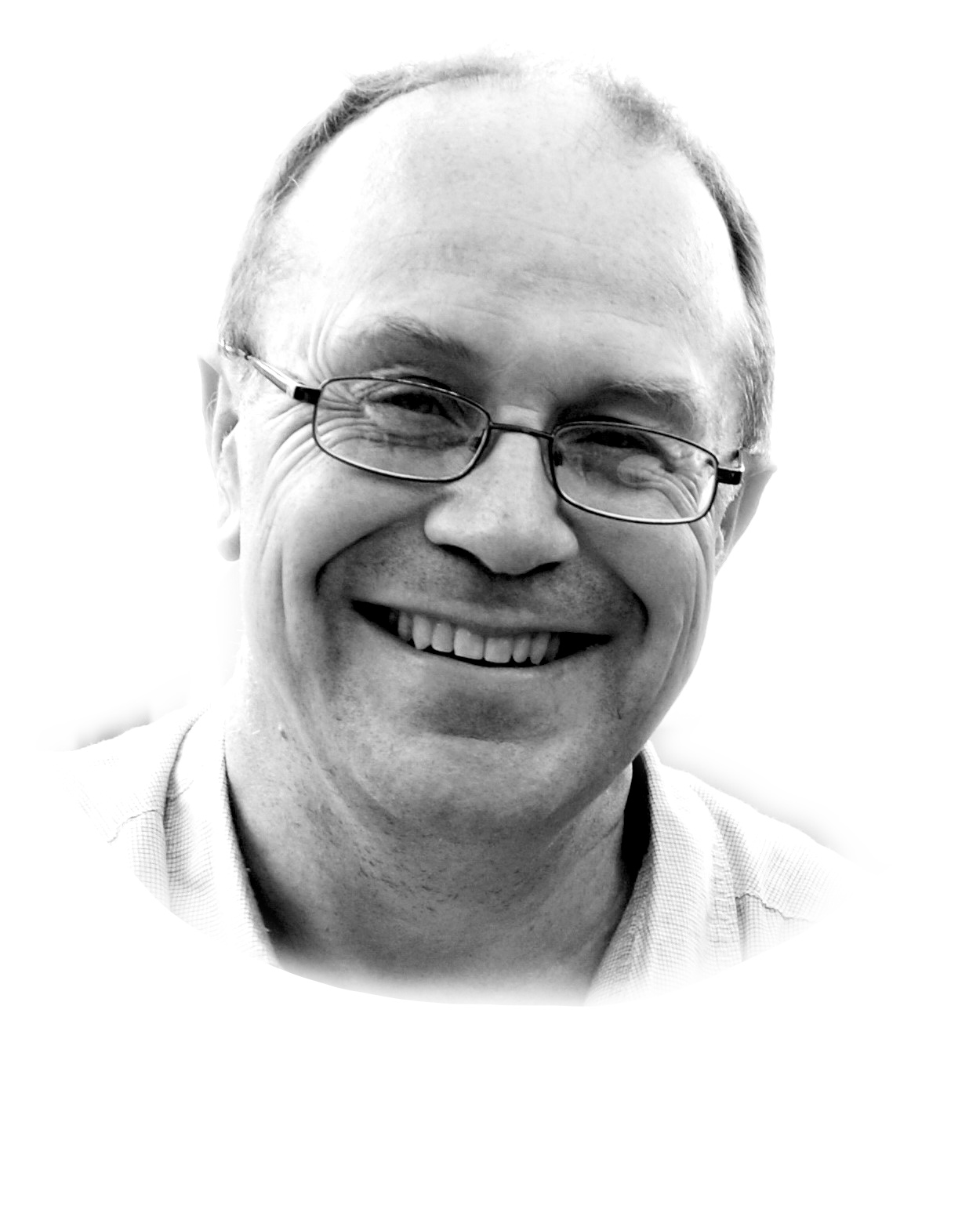
About Paul Marks
Paul is an award-winning journalist in London focused on technology, cybersecurity, aviation and spaceflight. A regular contributor to the BBC, New Scientist and The Economist, his current interests include electric aviation and innovation in new space.