Your Brain on DMT... - by Andrew R. Gallimore Your Brain on DMT...
Length: • 12 mins
Annotated by Zakhar
Zakhar: DMT has been shown to disrupt high level networks like the DMN, resulting in increased communication between networks and a "globally hyperconnected brain state". It also affects the limbic system, which sends information up to the cortex to help construct the world model. In addition, theta oscillations in the hippocampus have been seen to coincide with peak visual effects of DMT, suggesting that the experience is being modulated by information from subcortical structures.
A breakdown of Imperial College's new DMT fMRI/EEG study.
15 hr ago
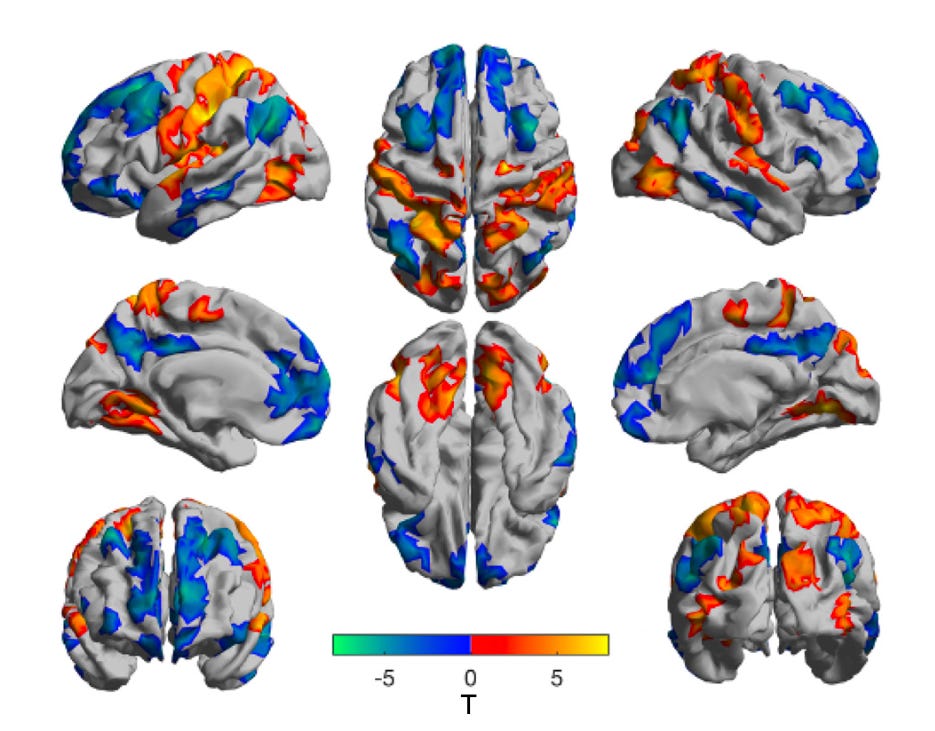
Recently, Imperial College London’s Psychedelic Research Group, led by Chris Timmermann, published the first major functional neuroimaging study on DMT which, like the previous papers published by the group on both psilocybin and LSD, provide important and novel insights into what’s actually going on in the brain when someone is given this most remarkable of psychedelic molecules.
Download the paper here: https://www.pnas.org/doi/10.1073/pnas.2218949120
Like all major neuroimaging papers, it’s both highly technical and covers a lot of ground. As such, I won’t attempt to explain all the technical details of the paper, which would require quite a bit of neuroimaging background to fully understand. Rather, I will concentrate on what I consider to be the main findings of the paper — what the experiments revealed about DMT’s effects on the brain and how this relates to the experienced effects, rather than the specific neuroimaging techniques that were employed. Also, I won’t discuss every single result in detail, but only what I consider to be the most revealing results.
If you haven’t already done so, I strongly recommend you first read my brief primer on the mechanism of action of the classic psychedelics:
Some content could not be imported from the original document. View content ↗
Or, even better, read my latest book Reality Switch Technologies, which will give you a solid foundation in the neuroscience of psychedelic drug action:
https://www.buildingalienworlds.com/books.html
However, if you haven’t read the book and don’t have some background in neuroscience, here’s some important basic information you’re going to need to understand what follows (and the paper if you decide to dig in yourself):
The Cortical Hierarchy.
The cortex is hierarchically structured, with the lowest sensory areas (primary visual and auditory cortex, for example) receiving information from the sensory apparatus. These areas are described as unimodal, in that they only process and represent information from a single sensory modality, and are responsible for processing and representing basic sensory information, such as lines, shapes, colours, and movement. These unimodal areas are connected to higher-order areas that integrate the information from the lower areas and then themselves communicate with even higher order areas. At the highest level of the hierarchy you reach the transmodal (or polymodal) areas that integrate information from all sensory modalities, as well as being important in cognition and uniquely human activities, such as language and complex thought.
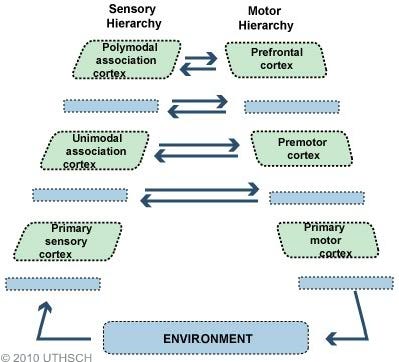
In the paper, the authors talk a lot about the “transmodal association cortex pole” — TOP — of the cortex, which refers to these evolutionarily new (and highly developed in humans) areas at the top of the cortical hierarchy. These areas are furthest from the immediate concerns of processing and responding to sensory information and more concerned with abstract cognition, self-reflection, and imagination. So we can see a gradient emerging, with the unimodal sensorimotor areas of the cortex at one (bottom) pole, closest to the environment, and the transmodal higher-order areas at the other (top) pole.
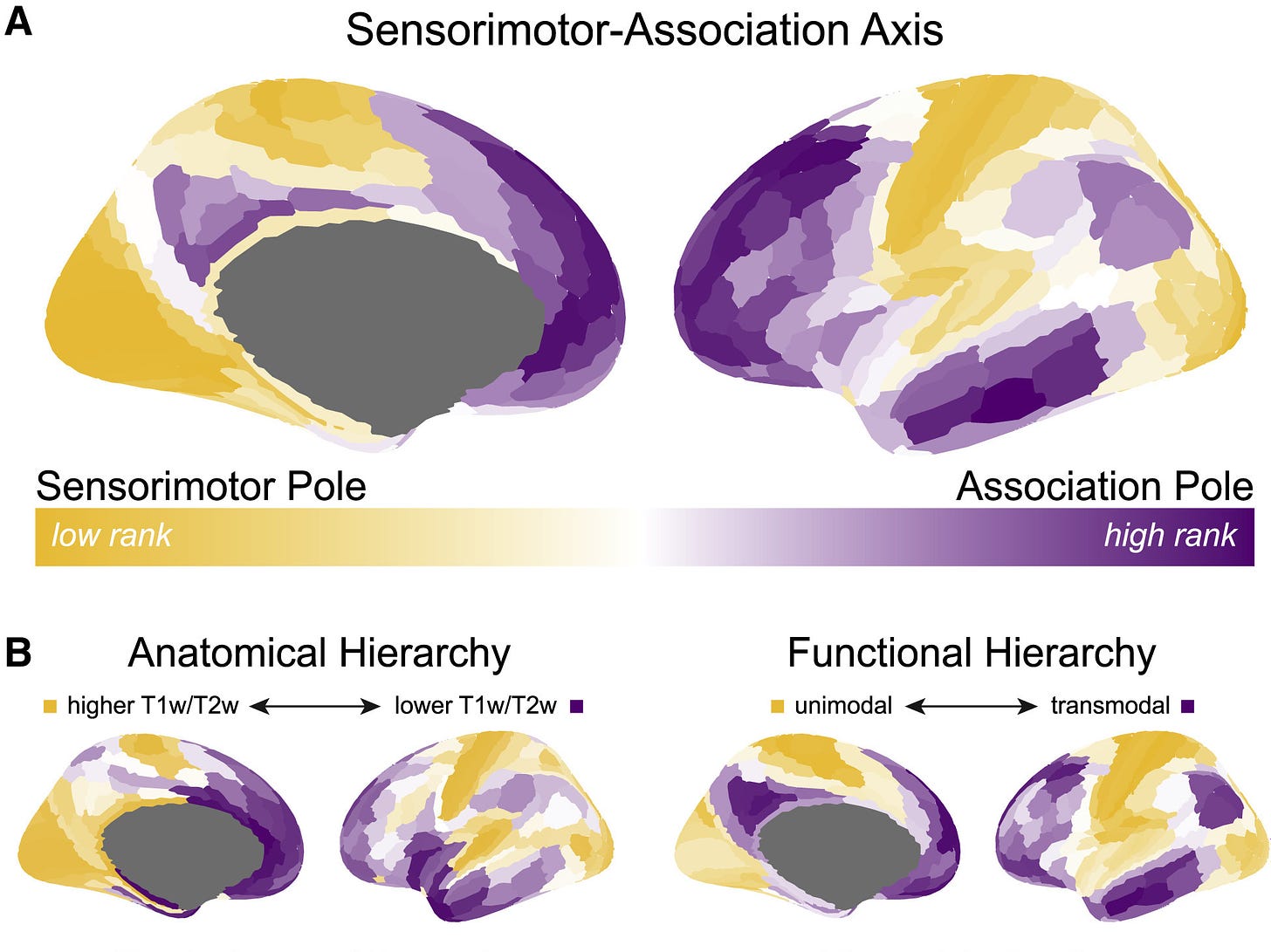
As discussed in the primer (again, read it: LINK), model predictions about the evolution of sensory information flow down the cortical hierarchy. Mismatches between predictions and actual sensory inputs generate error signals that flow up the hierarchy and are used to update the model until prediction errors decline. This is the fundamental basis for the brain’s ability to “filter” sensory information: only unpredicted (i.e. surprising) sensory information evades being extinguished by the inhibitory predictions and makes its way up into the higher levels of the cortex.
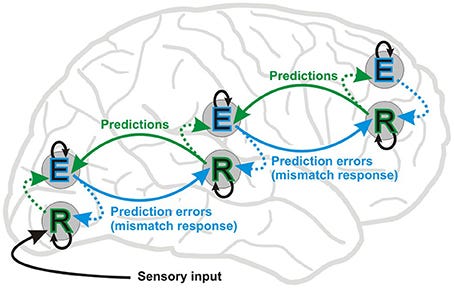
Connectivity.
Another oft confused term is connectivity, which obviously refers to the way neurons and cortical areas are connected to each other. However, there are three types of connectivity you need to be aware of:
1. Structural connectivity: This refers to the actual physical “wiring” of the brain, the synaptic connections between neurons.
2. Effective connectivity: This refers to the way activity in one cortical area causes an effect on the activity in another area. Of course, effective connectivity depends upon structural connectivity — activity in one cortical area cannot affect another area unless there is a connection, direct or indirect, between them.
3. Functional connectivity: This is the most fleeting form of connectivity and, whilst depending on structural connectivity, doesn’t refer to physical connections between areas but, rather, to connections in time. When, for example, the brain is encoding an object in the visual field, neurons in the areas that represent the features of that object are simultaneously active: those areas are functionally connected. So, functional connectivity is a transient temporal coincidence of activity in separate areas of the cortex. In a functional MRI image, these areas of the cortex are seen to ‘light up’ at the same time. Unlike structural connectivity, which can persist for a long time, functional connectivity can change from moment to moment as the cortex moves from state to state. This is the flow of moments, one state after the other, that is your experience of living in a world.
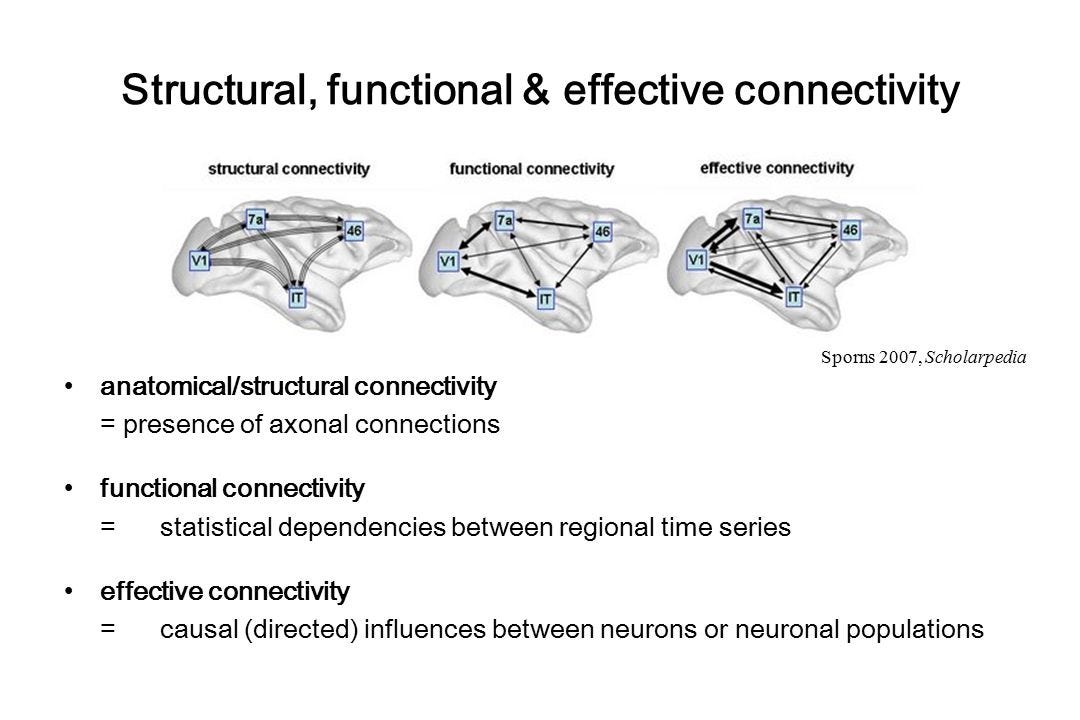
Whilst there are imaging techniques to measure structural and effective connectivity, most of the time you will read about functional connectivity in functional MRI studies. So, when you read that functional connectivity increased or decreased, this isn’t referring to structural changes in the brain but, rather, the rapidly shifting patterns of cortical activity.
High-order Networks.
Whilst the entire cortex forms a complex network of neurons and other cells, different areas of the cortex also form high-order networks that allow the brain to shift between more global states of activity. If we consider a single area of the cortex to be a network of neurons, then these high-order networks are networks of networks (or networks of cortical areas).
Three important brain area networks include the central executive network (CEN), the salience network (SAN), and the default mode network (DMN) (also called the task-negative network). The CEN is a network of brain areas activated during attention-demanding goal-directed tasks, such as solving a mathematical problem or writing a letter, and is important in holding and manipulating information in working memory. In contrast, the DMN is activated during daydreaming, when thinking about the future or reminiscing about the past, or just letting the mind wander, and is often considered the resting state of the brain (hence default mode network). The SAN helps the brain switch between the CEN and DMN according to demand. The CEN and DMN are anti-correlated, meaning they actively inhibit each other — when the CEN is active, the DMN is inhibited, and vice versa.
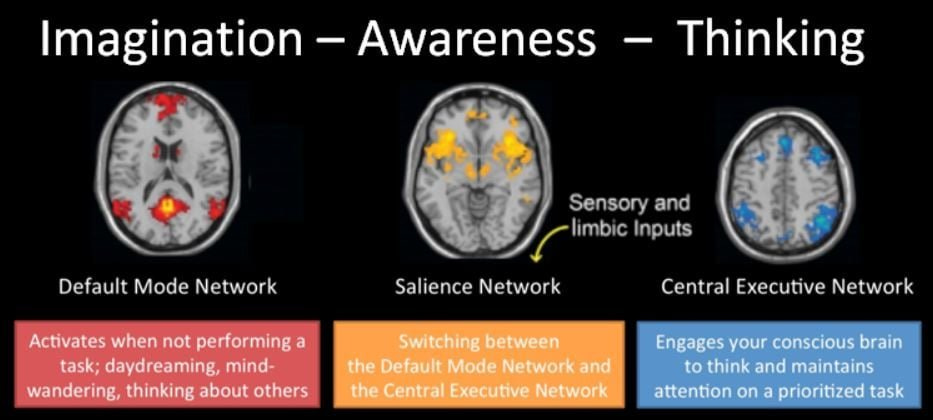
These networks of brain areas give the brain another level of control over its activity and the way information flows through itself. The ability of brain area networks to regulate other networks is important in this control, allowing activity to be controlled and focused on a particular task. Obviously, it’s difficult to focus on a cognitively-demanding task if you’re also daydreaming about your future career or who you might marry. As such, you will often read that these networks are segregated, meaning there’s strong functional connectivity between areas within the network (within-network connectivity), but lower functional connectivity to other networks (between-network connectivity). A high level of network segregation shows that the cortex has solid control over and is properly organising its activity. Since these high-order networks have broad control over large areas of the cortex, disrupting them can have consequences for the organisation of activity across the cortex (as we shall see).
Oscillations.
One of the most remarkable properties of the cortex and the other regions of the brain to which it’s connected is their ability to generate and synchronise oscillations at varying frequencies. Oscillations, observed at various frequencies using EEG, can entrain large groups of neurons that aren’t directly connected to begin firing in synchrony. Synchronisation is one of the ways activity can be coordinated across large areas of the cortex. In general, low frequency alpha oscillations are generated by the deep layers of the cortex and are markers of feedback activity (i.e. predictions) travelling down the cortical hierarchy (LINK). In contrast, high frequency gamma oscillations are markers of feedforward activity (i.e. error signals) propagating up the cortical hierarchy. When you close your eyes (and thus when you’re not actively viewing the world outside), alpha oscillation power increases and gamma power decreases, since fewer error signals are being generated. When you open your eyes again, alpha power drops and gamma power increases.
Maintenance of synchronised alpha oscillations depends upon a carefully regulated balance of excitatory and inhibitory activity (the E-I balance) in the deep layers of the cortex. The classic psychedelics stimulate deep neurons by activating 5HT2A receptors, disrupting this E-I balance and thus the generation of synchronised alpha oscillations. Desynchronisation of alpha oscillations and a reduction of alpha power is one of the most consistently observed effects of the psychedelics in human neuroimaging studies, reflecting disruption of the high-level world model and its predictions.
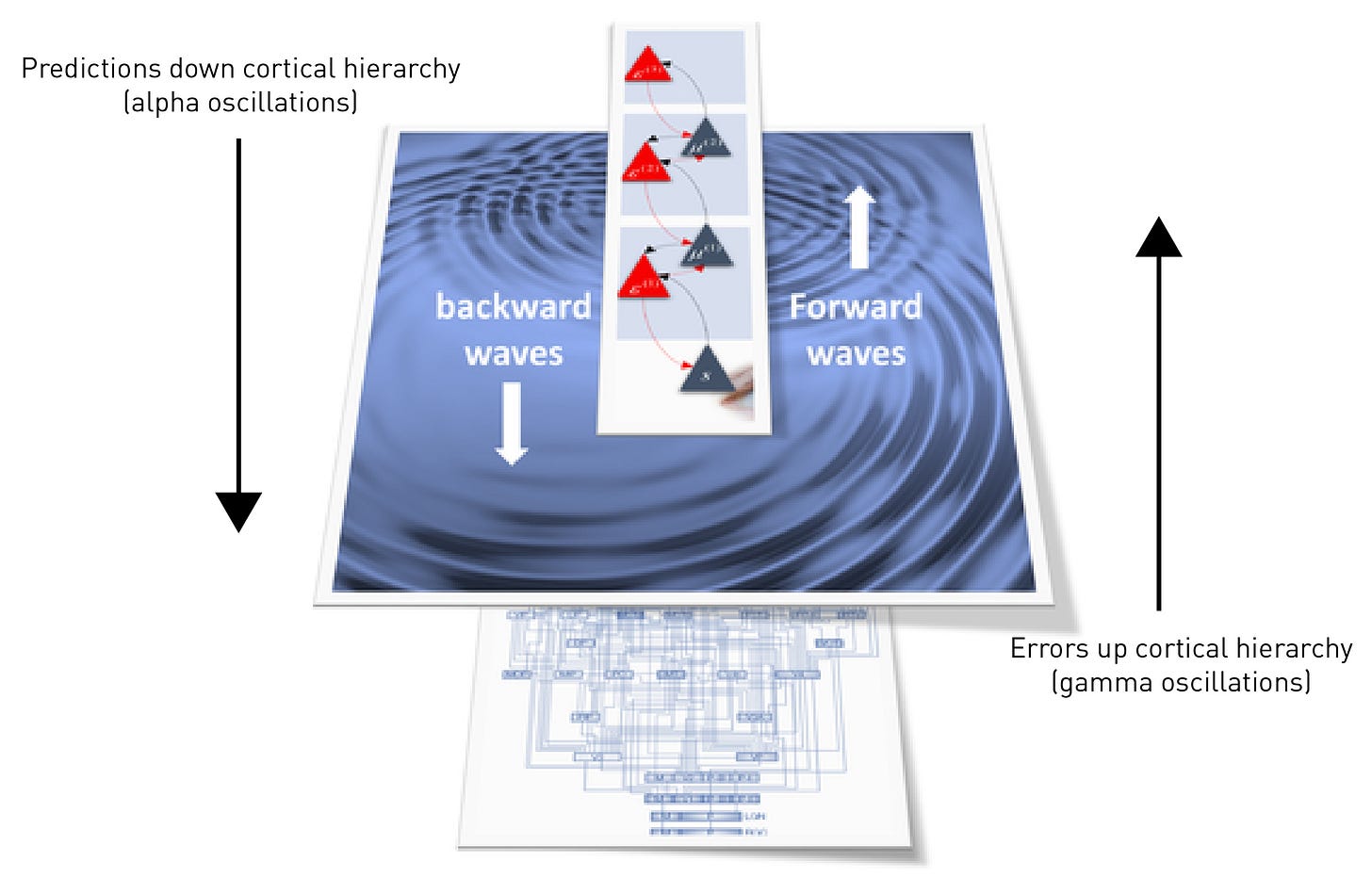
The Limbic System.
Sitting below the evolutionarily new neocortex are the much older subcortical regions, which include the so-called limbic system, as well as deeper brain areas such as the basal ganglia, controlling movement, and the brainstem, which controls basic unconscious processes required for life (such as heart rate and breathing). The limbic system includes areas such as the hippocampus, which is important in the formation of new memories, and the amygdala, which has a central role in the fear response. Broadly, it’s sufficient here to think of the limbic system as regulating memory and emotions.
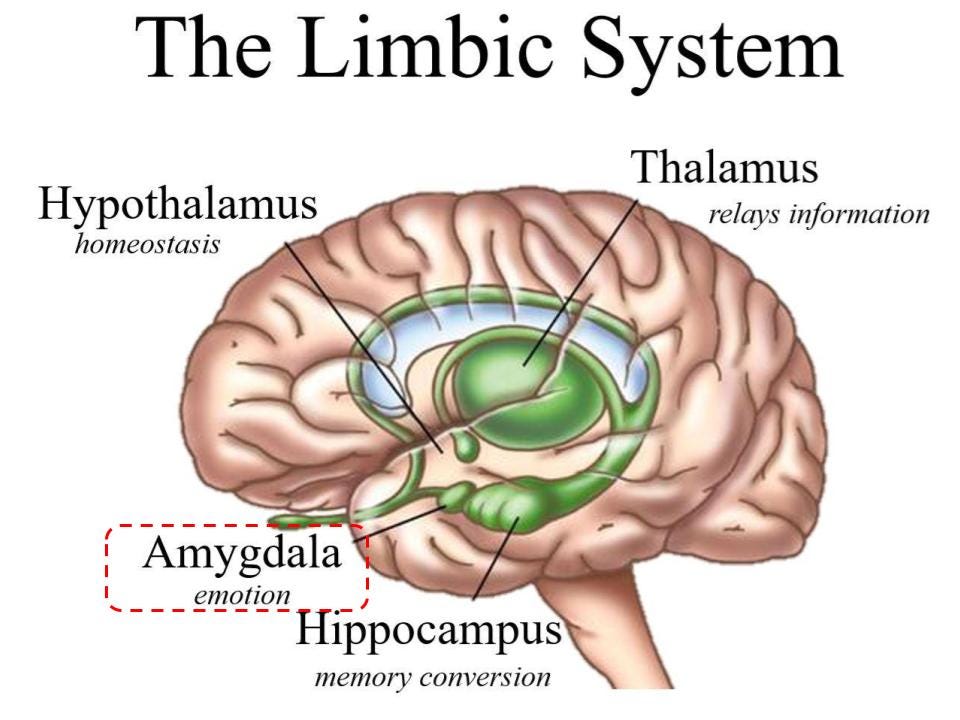
The Study...
OK, now to the DMT study itself.
Prior to this new study, a range of human functional imaging studies with psychedelics, mainly psilocybin (LINK) and LSD (LINK), have established some basic effects on cortical activity:
- Decreased within-network functional connectivity (FC), also referred to as “network integrity”. In other words, high-order networks, such as the DMN, are disrupted and become more disorderly.
- Increased between-network FC or decreased “segregation”. Networks that are usually well segregated from each other show more functional connectivity between each other.
- A globally hyperconnected brain state. On average, each cortical area shows greater functional connectivity to other areas — more cortical areas are “speaking to each other”.
Together these results reveal that the high order networks are disrupted and losing control over activity in other networks. Overall, cortical activity (and the world model it represents) becomes more disorderly and fluid, exploring a larger repertoire of states more freely.
Note that these observed effects are not independent and it’s helpful to try and think about how they relate to each other. For example, the reduction of alpha synchronisation and power mentioned earlier is a marker of this disorderly activity, which is further revealed by the decrease in functional connectivity (integrity) within high-order networks, such as the DMN. As networks break down and information begins to flow more freely between normally disconnected areas of the cortex, functional connectivity between networks increases, segregation decreases, and the “globally hyperconnected brain state” emerges. You see, it’s all connected (pun intended).
As in these earlier studies with psilocybin and LSD, DMT also disrupted high level networks, such as the DMN, increased communication between networks, and generally produced a more complex and fluid cortical state. In addition, the results revealed a “drug-induced collapse of the defining principal gradient of the brain”. In other words, there was reduced segregation and definition between the levels of the sensory processing hierarchy, further indicating loss of control of the higher over lower order areas.
Notice below how the integrity (within-network connectivity) decreases in the DMT state (vs placebo, PCB) in almost all the high-order networks, whilst global functional connectivity (GFC) increases:
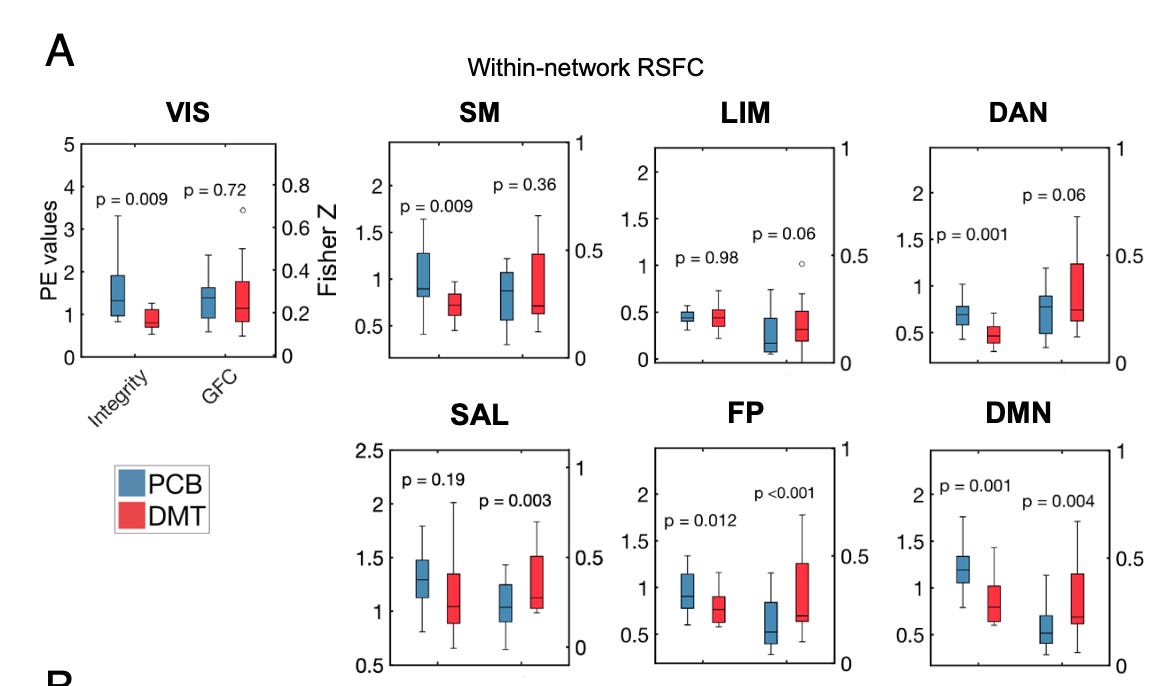
Don’t be concerned too much with how this ‘gradient score’ was calculated, but notice how the sharp definition between cortical areas is reduced between the placebo (PCB) and DMT state:
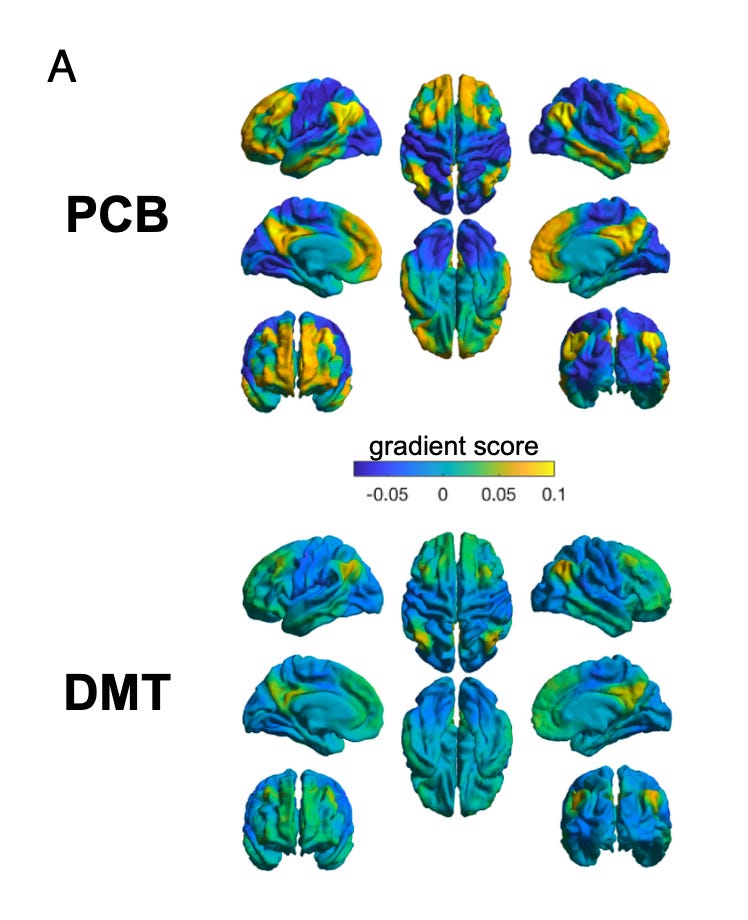
The densest population of 5HT2A receptors (those activated by the classic psychedelics, including DMT) are found in transmodal areas at the “TOP” of the cortical hierarchy, so this is where we should find the principal site of action of DMT.
What would you expect to happen if these areas were disrupted?
Well, the TOP of the cortex is responsible for generating high level model predictions that are propagated down the cortical hierarchy, having broad control over large areas of lower sensory cortex. So, we should see a reduction in synchronised (i.e. ordered) alpha waves travelling down the cortical hierarchy (backwards travelling waves) and, since these predictions are inhibitory (their role is to suppress the upwards flowing error signals), a concomitant increase in synchronised gamma waves (prediction errors) flowing up the cortical hierarchy (forward travelling waves). And, indeed, this was one of the major findings in Timmermanns’s first DMT study using EEG (LINK) and was replicated in this new study: at the peak of the DMT experience, backwards (downwards) travelling waves decreased and forwards (upwards) travelling waves decreased in a manner that was almost indistinguishable from a normal open-eye state when the subject was viewing the external environment. The difference, of course, is that the subject had his/her eyes closed and was viewing a quite different “environment”. Whether or not the brain is actually receiving sensory information from another environment in the DMT state is very much the million dollar question. Most neuroscientists would answer in the negative. I remain agnostic.
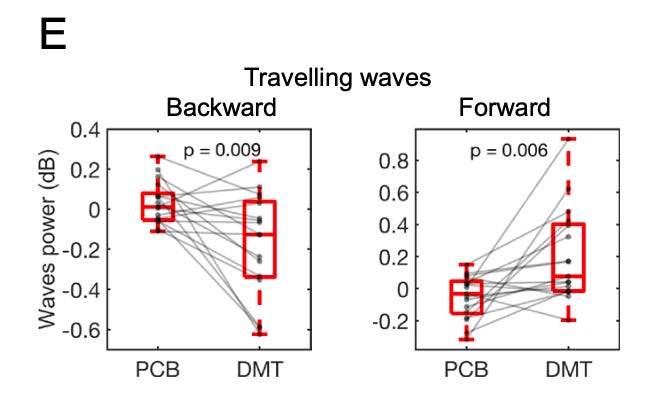
For me, one of the most intriguing results, again implicating the TOP of the cortical hierarchy and the higher-order cortical networks such as the DMN, was the effect on the limbic system. It’s useful for our purposes here to think of the world model constructed at the highest levels of the cortical hierarchy as receiving information from two directions: from the environment via the lower order sensory cortices in the form of error signals, and from the subcortical regions, including the limbic system.
So, your experienced world is informed not just by sensory information from the environment, but also memory and emotion (and endogenous sensory information from your body, but we’ll leave that for now). Of course, this makes perfect sense: you’ve spent your entire life building memories (and their associated emotions) from your experiences of the world. So it’s not surprising that these memories turn out to be extremely useful in modelling the world in the present moment. As such, there’s always a flow of information from these subcortical regions up into the cortex where your world model is constructed.
However, at the same time, it's essential that, just as with sensory information, the cortex keeps this flow of information from the limbic system in check. If your experienced world is to represent a stable and functional model of the environment in the present moment, it’s not a good idea to have memories and emotional material flowing untrammelled into the cortex. As well as attempting to stem the flow of sensory information from the environment, the cortex must also regulate the flow of information from these subcortical regions.
So, again, what would we expect to happen if these high-order cortical areas were disrupted?
Well, we’ve already seen that this leads to an increased flow of sensory information (error signals) from the lower sensory areas. We should also then expect to see an increased flow of information from the limbic system. And, indeed, this is what we see — and not for the first time. Back in 1956, a study showed increased activity in the medial temporal lobes (where the hippocampus is located) after both LSD and mescaline administration, measured using electrodes implanted in the brain (LINK). And, much more recently, Franz Vollenweider’s group showed (using PET imaging) that the largest increase in neural activity following LSD administration was in the temporal lobes, which correlated with drug-induced visual imagery (LINK).
It’s tempting, but entirely inaccurate, to imagine that the complex imagery experienced in the DMT state is flowing from the hippocampus and other subcortical regions. But there are no complex images in these regions. The hippocampus doesn’t contain your memories, it simply reactivates cortical patterns that represented the images in the first place. Think of the hippocampus as a “memory index” that points to particular patterns of cortical activity that the brain, in the past, judged to be of value (emotion is important here, since experiences accompanied by strong emotions are likely to be worth remembering — this is why the hippocampus is strongly connected to the subcortical regions responsible for emotional processing, such as the amygdala). At most, the hippocampus contains a “summary sketch” of the neocortical representation — the image/experience — that it reinstates (LINK).
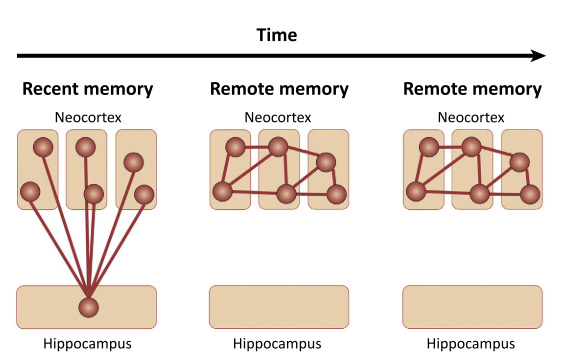
During REM sleep, the hippocampus is particularly active in reinstating these representations, which is generally thought to be important in their consolidation and maintenance as long term memories in the cortex. Interestingly, Timmermans’s first DMT study (see above) observed an increased in theta oscillations (the frequency at which the hippocampus operates) coinciding with the peak of the visual effects, suggesting that the experience was being modulated by information from these subcortical structures. However, I feel we must be careful with the conclusions we draw from this.
If disinhibition of limbic and other subcortical structures by disruption of high order cortical networks was the primary driver of DMT imagery, we should expect DMT visions to be much more like dreaming. And, generally, dreams are pretty dull and continuous with the waking world, albeit with a high propensity for negative social interactions and their concomitant emotions. DMT visions are anything but, and much more likely than not to bear no resemblance whatsoever to the normal waking world, nor indeed the dream world. If you’re looking for machine elves and hyperdimensional alien intelligences, I’ve trouble believing you’re going to find them in the hippocampus or in the deeper and more ancient subcortical regions of the brain. This explanatory gap has yet, in my opinion, to be traversed.
So, do I think DMT visions are informed by subcortical structures? Most definitely. All visual experiences are. Do I think the flow of information from these structures explains the inordinately complex and novel imagery characteristic of the DMT state? I’m not convinced.
Recommend Alien Insect On Drugs to the readers of A bowl o'pasta
I write about the the science and culture of psychoactive drugs, neuropharmacology, and consciousness.
Recommend Alien Insect On Drugs
I write about the the science and culture of psychoactive drugs, neuropharmacology, and consciousness.
By Andrew R. Gallimore · Launched 5 months ago
Why are you recommending Alien Insect On Drugs? (optional)
Recommendations are shown to readers after they subscribe to your publication. Learn more